Design of Glass
DESIGN OF GLAZING AND SELECTION OF TYPE OF GLASS
The selection of type of glass involves consideration of a range of factors and combination of a number of processes to achieve the required set of properties. In some cases the design will be constrained by the limits of one or more processing steps, particularly when it comes to panel size, and the inner and outer panes of insulating units are commonly different types. Only after the glass type has been selected can a suitable thickness be calculated. In the UK, the current standard for this is BS 6262.
In many structural applications of glass there is no agreed method or precedent for design and in these instances an approach based on first principles is necessary. The fundamental approach is to anticipate scenarios in which the glass will get broken and provide levels of redundancy by duplication or by an alternative load-path that does not rely upon the integrity of the glass.
Design guidance, references to standards and built details for a wide range of structural glass applications is contained in the Institution of Structural Engineers guide Structural use of glass in buildings (I Struct E, 1999).
DEFLECTION LIMITS FOR GLAZING
There are no mandatory requirements for deflection limits on window glass specified in codes of practice commonly used in the UK, US, Australia and mainland Europe.
Deflection Criteria
Insulating glass unit (IGU) edge seals can be overstrained if the edges are allowed to deflect significantly, which leads to the ingress of water vapour into the cavity, premature internal ‘misting’ and early unit breakdown. Some manufacturers have robust edge seal constructions that will accommodate deflections of up to span/50 at full design wind load.
The response of glazing to hand pressure seems to be acceptable if its natural frequency is above 4 Hz, whereas glazing with a natural frequency of around 2 Hz feels flimsy because it wobbles readily if you push it.
Guidance from Standards
Most glass design codes allude to the need to limit deflection, but avoid direct specification. The UK codes for patent glazing (BS 5516) and the structural design of aluminium (BS 8118) set the following limits for mullion deflection:
- span/125 for single glazing
- span/175 for insulated glazing.
This deflection limit for single glazing limits the stress in the supported edges, which is important for annealed and wired glass. The limit for double glazing is intended to protect the edge seals of IGUs. The deflection limits also tend to be applied to the unsupported edges in one-way spanning glass.
DESIGN STRESSES AND LOAD FACTORS
There has historically been a lack of consensus regarding glass design stresses, with varying views held between industry and consulting engineers, and also across the continents, where the basis of design codes varies widely. There is no current UK code of practice providing a general basis for glass design.
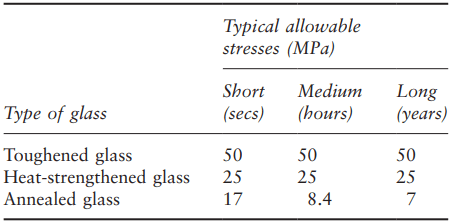
glass according to duration of load.
The permissible stresses in Table 1 have been used without load factors as general rules of thumb, but would not be appropriate for precise design of critical glass elements. Progress has been made on limit state design of glass, although the combination of loads of different duration combined with the time-dependent strength of glass makes it important to account for the stress history that is expected for a structural glass element, and so the combination of load factors becomes complex.
Haldiman et al. (2008) have published on behalf of The International Association for Bridge and Structural Engineering a structural engineering document on the structural use of glass, in which this subject is covered in great depth. This is an effective state-of-the-art work for practising engineers.
Strength of Laminated Glass
The strength and stiffness of laminated glass depend on the extent to which the interlayer couples the two sheets of glass in shear. If the shear transfer between two sheets is fully effective, they will behave as one, with thickness equal to the two combined.
However, plastic interlayer materials are viscoelastic to varying degrees, so they tend to relax and flow when stressed. At low temperatures or when loaded for a very short time, a viscoelastic interlayer may appear rigid and a laminated pane may be almost as stiff and strong as a monolithic pane of the combined thickness. This is known as ‘composite’ action.
At higher temperatures, or when loaded for a longer period, the individual plies slide over each other, allowing the deflection and bending stress in the glass to increase, which is known as ‘layered’ action.
WINDOWS
Standards for the design of glazing for windows exist in most developed countries, although there is wide variation in the methods of calculation and the values for the strength of glass used when calculating the required thickness. Large window panes tend to deflect by two or three times their own thickness under full design wind load, so membrane stresses are generated, being tensile in the centre of the pane and compressive around the edge.
These stresses act in combination with the bending stiffness of the glass and can significantly reduce the deflection and maximum principle stress compared with what is predicted by simple, first-order, bending theory. Large-deflection theory solutions to the bending of plates are more complex to calculate but some design codes, such as ASTM E-1300, provide reference charts to save the designer lengthy calculations.
Some other codes use simple bending calculations but factor up the design stress to allow for the fact that the calculated stress will be overestimated. This is one of the reasons why design stresses from window codes cannot be taken to be safe values for more general application to glass engineering.
Design of Insulating Units
When two panes of glass are sealed around their perimeter to create an insulating unit, the fixed mass of air between them obeys the General Gas Law (pressure × volume/temperature = constant). Consequently, the pressure inside the unit will rise if one pane is displaced because it changes the volume of the cavity (Fig. 1).
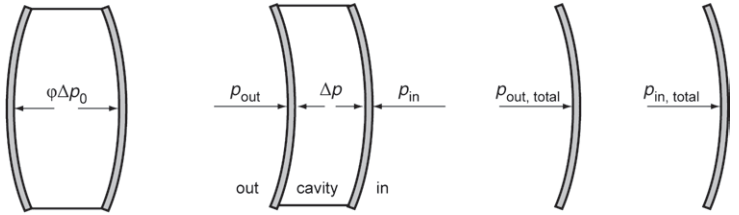
The wider the cavity, the larger the initial volume contained and the less significant are small changes in volume caused by displacement of the panes, so units with thinner air spaces will share load between panes to a greater degree.
When the temperature of the enclosed gas is raised, for instance in hot weather, the internal pressure rises and the panes of glass flex outwards, and in cold weather the effect is reversed. The pressure changes caused by temperature, atmospheric pressure and a change in altitude are considered together as the ‘climatic load’ on an insulating unit by some windowdesign methods.
Climatic loads are likely to be insignificant for large flat units with modest cavity width, because the glass deflects easily and the sealed volume changes rapidly, so the differential is equalised. However, climatic loads can become important to consider along with other loads if the units are very long and narrow, or curved.
GLASS WALLS AND STRUCTURAL GLASS ASSEMBLIES
The use of glass as a structural element in a wall is invariably linked to the desire for transparency or translucency. Glazed areas can function as collectors or dissipaters of energy under different weather conditions. The gain or loss of energy may not be beneficial at all times and glazed walls are increasingly required to be double glazed and incorporate selective coatings to control the transmission of incoming solar radiation and the loss of heat from the building.
The use of double-glazed panels and coated glass adds to the challenge of using the glass safely and reliably in a structural role. Glass in a wall could present a safety hazard in two ways if it should break and fall from the opening; it could injure someone below and it could allow someone inside the building to fall out.
Given the availability of heat-treated and laminated glass, it is reasonable to apply the criterion that if falling glass would present a danger to people then glazing should retain a reasonable level of stability after breakage, at least sufficient to allow further measures to be taken before any glass should fall.
In regions subject to hurricanes the most onerous requirements are placed upon glass, such as impact damage from a baulk of timber followed by several thousand reversing load cycles.
SKYLIGHTS
The criteria for glass roofs fall between the requirements for walls and floors; in some cases the roof of a building has full public access and is effectively a floor. However, most glass roofs will need pedestrian access for cleaning and maintenance and, even if safe routes are provided to avoid walking on the glass, it is essential to avoid the hazards associated with fragile roofing.
The Centre for Window and Cladding Technology in Bath has published a technical note, TN42, which gives guidance on glazedroof design and sets out a test regime to verify that the glazing will prevent someone falling through, even if the glass is broken (CWCT, 2004).
FLOORS AND STAIRS
The use of glass as a floor introduces the need for slip resistance and increases the level of robustness required. It also puts glass into a location that invites rough treatment. In a floor application the loading may remain applied for long durations, which means reducing the allowable stress according to the heattreatment condition of the glass being used and taking account of the viscoelastic properties of the polymer interlayers that will affect the degree of composite action between panes (Smith and Dodd, 2003).
GLAZING FOR SECURITY
Security against manual attack, ‘anti-bandit’ or ‘anti-break-in’ glass is intended to delay or prevent someone breaking through the glass with a variety of tools. The design objective is to absorb energy from the impacts of something like a hammer or an axe and avoid the interlayer being cut, torn or penetrated such that it would allow someone to gain access through the window. Glazing to resist ballistics is classified according to tests in which weapons of different calibre are fired at glass samples, usually in a group of three shots in a triangular pattern. The kinetic energy imparted by some weapons requires many layers of glass and interlayer, which results in a thick and heavy construction.
Polycarbonate sheet is often laminated in place of some glass layers, using polyurethane interlayer because of its potential to absorb energy. When glazing is exposed to a blast, the primary design objective is to protect people inside the building from injuries caused by flying glass. The broken panel must be kept attached to the frame if it is to prevent fragments flying across the room, so the glazing details have to be deep and robust.
The frame construction and its attachment to the building structure also have to be designed to carry the reactions from blast loading, which are sometimes calculated using non-linear analysis of the dynamic response of the whole system.